Tiny tumour tissues to fight cancer: A model case study for interdisciplinary science
Associate Professor Daniela Loessner occupies two worlds within Monash – engineering and medicine, specifically tissue engineering and cell biology.
Her work, at a tiny, tiny scale, is helping pave the way for more precise drugs to fight cancer by building three-dimensional models of tumour tissues.
The new review she led of the emerging tissue engineering field is outlined in Nature Reviews Materials, and shows that advances in several complex scientific frontiers provide highly accurate, 3D models of an individual patient tumour in which treatments can be tested.
The paper’s introduction explains that despite cancer being “the leading cause of death worldwide”, development of new treatments is in part hampered by wide variability. For example, the same type of pancreatic tumour is different between two patients, and there are differences within a tumour within a patient.
More than cell biology
The science also requires more than cell biology. It needs expertise in engineering of materials from synthetic and natural origin – polymers, algae, gelatine and cellulose. Specialist engineers also build bioprinters and use so-called “bioinks” to 3D-print the scaffolding for the 3D models.
Associate Professor Loessner holds her joint appointments in the Faculty of Engineering and the Faculty of Medicine, Nursing and Health Sciences, and also at the Leibniz Institute of Polymer Research in Dresden, Germany.
She’s an international expert on tumour tissue engineering, starting as a cell biologist in ovarian cancer and branching out into bioengineering, materials science, nanotechnology, and stem cell engineering – a case study for modern interdisciplinary science.
“Cell biologists can grow cancer cells in the laboratory, but we can’t understand tumours by growing cancer cells in isolation,” she explains. “The secret to designing better 3D cancer models is multidisciplinary collaboration between tissue engineering and biology.”
Read more: Genetic secrets of almost 2700 cancers unveiled by landmark international project
The 3D models are very small, about five millimetres in diameter. The base “matrices” and scaffolds are made of different biomaterials.
The innovation now is that the 3D models capture interactions between many cells found within a tumour tissue. Scientists can now look at the whole tumour microenvironment, with malignant and healthy cells inside, and the extracellular matrix outside.
“This matrix changes when a person develops cancer,” she says. “It becomes denser and stiffer, the composition changes, and then the tumour evolves, which helps the tumour to grow and also, unfortunately, the disease to progress.
“So the cell-matrix interplay has a large role in metastases,” she says, “and most people diagnosed with cancer die of metastases, not because of the primary tumour. We’re starting to know much more because we have these new technologies – we are trying them to use different new drugs to soften this matrix.
“If that’s what the patient receives first, and then you're hitting them with the chemotherapy, that should be much more effective.”
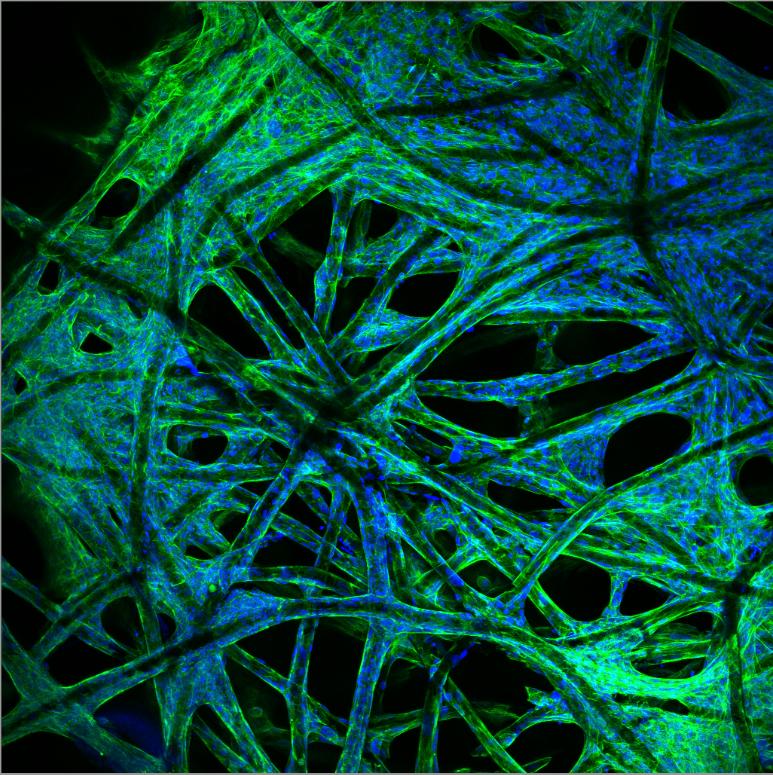
Communication is the key
Much of the behaviour of tumours and responses to treatments are now being linked to the communication between cancer cells and non-cancer or “stromal” cells, to the point where stromal cells supporting the tumour’s growth may themselves be targets for anti-cancer therapies.
Cancer cells can also secrete and remodel their own extracellular matrix, changing the physical properties of surrounding tissues in a way that may enhance cell invasion.
“For example, pancreatic tumours are very hard or stiff,” says Associate Professor Loessner, “and that hardness or stiffness can be associated with more aggressive disease that’s much more difficult to treat.”
Associate Professor Loessner and her team are also finding and implementing ways to make the stiffness and elasticity of the 3D models “tuneable” so they can mimic the physical properties found in tumour tissues at different stages of disease progression.
"By replicating the physical environment, we can get cancer cells to grow in the laboratory just as they would in their original setting in the body,” she says.
Less reliance on clinical testing
The better the tissue-engineered 3D model, the more closely scientists will be able to study and understand cancer cell growth, migration and invasion, and observe responses to chemotherapies and the rapidly developing range of immunotherapies or stromal-targeting therapies.
The scientific advances mean less reliance on pre-clinical testing with an aim of “co-clinical” treatment or “co-clinical” trials where a patient and a laboratory model of the same tumour tissue are treated and compared. Not in real time as yet, but very quickly – in about three to four weeks.
“We get a quick response, we go back to the patient and the oncologist, and recommend a specific treatment combination for that patient.
The paper says two research areas particularly in need of new 3D oncology approaches have “unmet clinical need” – pancreatic tumours, which are forecast to be the second-leading cause of cancer-related deaths within this decade, and rare paediatric cancers such as neuroblastoma, osteosarcoma and Ewing’s sarcoma.