How do ecosystems collapse? Our study shows evolution plays a role
Blake
Dying coral reefs, rainforests transforming into savannas, grasslands turning into deserts – these are ecosystem “tipping points”, boundary lines we’re desperate not to cross.
In dynamic systems filled with life, these critical thresholds aren’t set in stone. Since organisms can evolve, the tipping points within these ecosystems might evolve, too.
Most of us think of evolution as a glacial process, too slow to witness in a single lifetime. But evolution, especially in the microbial world, can happen very quickly. Consider antibiotic-resistant bacteria that emerge within years, or the COVID-causing virus evolving new variants in mere months.
When the conditions are just right, evolution can go into overdrive – although that’s usually not good for us.
Our latest research, published in Nature Ecology and Evolution, reveals the first experimental evidence that tipping point behaviour can indeed evolve, and evolve quickly. This raises an exciting prospect: Could understanding the evolution of tipping points help us steer ecosystems away from collapse?
Equilibria, diversity and exclusion
Tipping points are critical thresholds where a small change in environmental conditions can lead to a dramatic and often irreversible shift in an ecosystem’s state. But what exactly does this mean?
An ecological community is a network of interacting species – plants, animals and microorganisms – that live in the same area and are interconnected through various relationships such as predation, competition and symbiosis.
A healthy ecological community has a balanced mix of species that perform essential roles. They contribute to services such as pollination, nutrient cycling, water purification, and climate regulation.
When an ecosystem is pushed toward a tipping point due to stressors including climate change, habitat destruction or pollution, this balance is disrupted.
Crossing these thresholds often leads to less diverse communities that fail to perform essential functions. This results in species extinctions and a loss of vital ecosystem services.
Take coral reefs, for example. Corals have a symbiotic relationship with microscopic algae called zooxanthellae. Rising ocean temperatures push the ecosystem toward a tipping point – a critical thermal threshold beyond which corals expel the algae in a process known as coral bleaching.
If the stressful conditions persist, the ecosystem shifts from a vibrant, biodiverse reef to a barren underwater landscape. This can lead to the collapse of the entire reef ecosystem and the loss of marine life that relies on it.
So, we know tipping points are crucial for us to understand. But observing and mapping tipping point behaviour in these complex systems is extraordinarily difficult.
To explore how evolution might influence tipping points, we turned to the microscopic world.
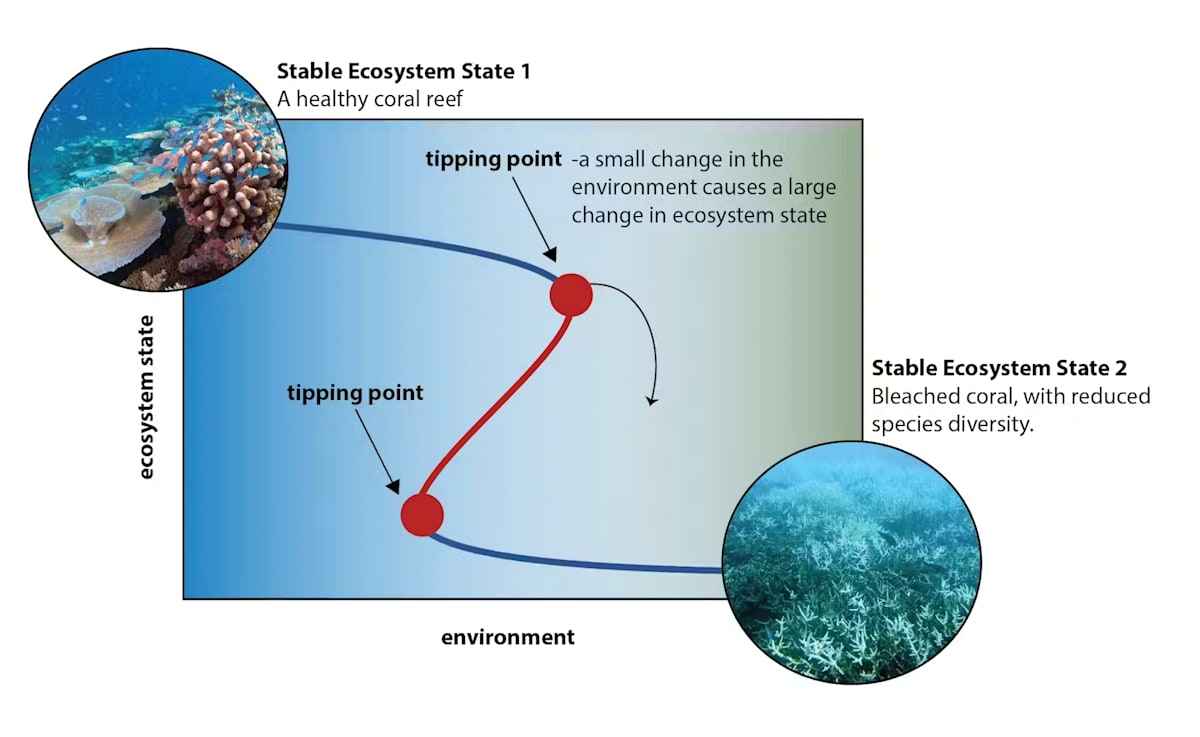
Evolution in real time
Bacteria reproduce at astonishing speeds, allowing us to witness evolution as it happens. Over just 400 days, we guided a model microbial community of E. coli through 4000 generations. This would be equivalent to about 100,000 years for humans.
One of the unique perks of working with microbes is our ability to freeze them, like biological snapshots in time. This means we can store the original “ancestral” community, and later revive it to compare with its evolved descendants.
By exposing both the ancestral and evolved communities to a range of environmental stresses, we could observe when they remained stable and when they collapsed.
We discovered that evolution can cut both ways.
As species co-evolved to become better-adapted to benign conditions, they became more sensitive to stress. This had no impact until we exposed the community to adverse environmental conditions. This triggered unexpected destabilisation, bringing the tipping point closer, and causing collapse sooner than anticipated.
On the flip side, when we specifically evolved the community to withstand environmental stress, the species adapted in ways that allowed them to coexist under much harsher conditions. This effectively delayed the tipping point.
Md Ariful Islam/iStock/Getty Images Plus
A new model
To illustrate these ecological changes, we developed a mathematical model that can allow for tipping points.
By tweaking key factors such as competition levels, stress resistance and species growth rates, we could simulate how different evolutionary changes affect an entire ecosystem’s trajectory.
If we want to forecast the stability of ecosystems, having a way to include evolution is a significant step.
On the one hand, our results indicate that “directed evolution” – with humans helping species adapt to environmental changes – could bolster ecosystem resilience and prevent collapse.
On the other hand, our research warns us evolution isn’t always a saviour. For example, evolutionary changes that increase the dependence, or impact, of one species on another species may speed up destabilisation, making tipping points arrive sooner than we’d like.
As we grapple with unprecedented environmental challenges, understanding evolution’s role in ecosystem stability becomes more critical than ever. Just as evolution can help harmful bacteria outsmart our antibiotics, it can also shift the tipping points of entire ecosystems, for better or worse.
This article originally appeared on The Conversation.
Monash is pioneering a path to a greener, smarter, more equitable and sustainable future, where emissions are lower, and the natural environment and humans thrive. We look forward to participating at COP29, where we aim to accelerate global action on sustainability, empowering diverse voices from across the Indo-Pacific and influencing superior policy outcomes across a broad range of issues. Find out more monash.edu/cop29
About the Authors
-
Christopher blake
PhD Candidate, Faculty of Science
Christopher is an evolutionary microbiologist conducting his PhD at Monash University with Michael McDonald. He’s mainly interested in how complex microbial communities evolve together and adapt to rapidly changing environments. His research focuses on how evolution can alter the stability landscape of microbial communities, both as free-living communities and within host systems.
-
Mike mcdonald
Associate Professor, School of Biological Sciences Centre to Impact Antimicrobial Resistance
Mike established his independent lab in the School of Biological Sciences at Monash University in 2016. He joined the Centre to Impact Antimicrobial resistance as a founding member in 2020, and currently leads the training and inclusion working group to develop the next generation of AMR experts. His research focuses on the genetics of adaptation. To study this question, his lab propagates populations of yeast (and other microbes) for thousands of generations in a variety of laboratory environments.
Other stories you might like
-
The cruel inequality of climate change-induced disasters
People living with disability are disproportionally affected by climate change-induced disasters, which is why we need more disability-inclusive decision-making in climate adaptation plans.
-
The human health impact of climate change
The world has talked at great lengths about how climate change is an environmental crisis. But what about the human health effects that come from it?
-
Youth leading the charge: Transforming food systems for a sustainable future
Facing a triple-planetary crisis, our ecological systems need a shift to circular economies. Youth can drive sustainable food systems through mindful choices.